- About Terumo
- Business Overview
- Sustainability
- Technology
- Investors
- News
- Corporate
- Corporate
- Financial
- Global Network

This article is not intended for users in Japan.
For those who are accessing from Japan, please visit the following site.
https://www.terumo.co.jp/technology/stories/
Cancer has been called the "emperor of all maladies". Liver cancer is among the most common cancers around the world and has one of the highest mortality rates. According to a 2020 WHO survey, liver cancer is the third leading cause of cancer death behind lung and pancreatic cancer. Innovative therapies are needed to offer patients suffering from cancer a better future.
Surgical resection has long been the only treatment option for early liver cancer, offering a change of long-term survival and cure. As the cancer advances, however, resection becomes increasingly difficult. Locoregional therapies such as ablation (a method of locally destroying tumor tissues through changing temperature), transarterial chemoembolization (TACE, a method of locally delivering chemotherapy and depriving tumors of nutrients) or radioembolization can be the most optimal treatment options when resection (or transplantation) is no longer an option.
Radioembolization, also known as selective internal radiation therapy (SIRT), is a targeted therapy for treating liver tumors. Radioembolization makes use of the phenomenon that tumors in the liver receive their blood supply primarily from the hepatic artery, whereas blood supply to the healthy liver is primarily provided by the portal vein. During a radioembolization procedure, millions of radioactive microspheres, are administered by a catheter directly into the hepatic artery (Figure 1). Due to the preferential arterial blood flow towards the liver tumor(s) and the specific size, shape and density of the microspheres (mean diameter of 30 micron), the microspheres primarily accumulate in and surrounding the liver tumor(s) (Figure 2). Subsequently, tumor cells are killed by the radiation dose which is delivered locally. With radioembolization, a relative high radiation dose is delivered to the tumor(s) whereas the healthy liver tissue remains largely spared.
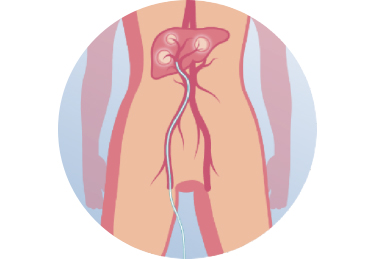
Figure 1. The microspheres are administered to the liver using a catheter which is placed in (distal branches of) the hepatic artery
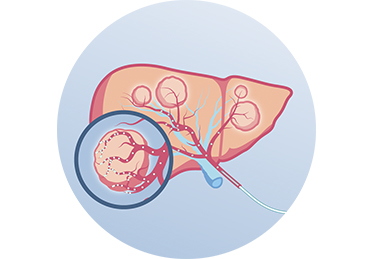
Figure 2. After administration, the microspheres are transported by the blood flow to the vascularized tumor(s) where they lodge in and around the tumor to emit a targeted irradiation dose.
Radioembolization with holmium-166 microspheres
Improving radioembolization by using imaging
One of the key challenges in radioembolization is to predict the distribution of microspheres, and thereby the therapeutic radiation dose to the tumor. Having insight in the microspheres distribution, and thus the dose distribution, is important, as clinical studies have shown that radioembolization is most effective if tumor(s) receive a sufficient radiation dose. The first isotope used for conventional radioembolization for the last decades, Yttrium-90, however, lacks properties which allow easy and accurate visualization. Conventional radioembolization is therefore often seen as a black box, with limited feedback on or control over the distribution of microspheres in the liver.
This need for an 'imageable' microsphere was already identified by the clinical team at the University Medical Center Utrecht (UMCU) in the Netherlands in 1994. At that time, the team started a search for an isotope that would have comparable therapeutic potential as Yttrium-90, while having improved imaging features. The microspheres that were developed by the UMCU are based on the isotope Holmium-166, which does have properties enabling accurate visualization of the microspheres and their distribution after administration. The holmium-based, imageable type of microspheres furthermore enabled the clinical team to use a small 'test dose' to verify dose uptake in the tumors prior to treating a patient with a therapeutic dose. Lastly, it allowed verification of the therapeutic treatment, ensuring that the treatment is performed as planned, also enabling the possibility to verify that the intended dose ends up in the tumor, instead of in surrounding – healthy – tissue, confirming the safety of use.
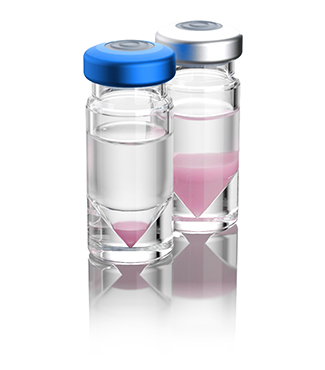
Holmium-166 Microspheres
(Radiation-emitting microspheres, Left: test dose; right: treatment dose)
The safety and efficacy of radioembolization using holmium-166 microspheres has been established in various clinical trials. Results from trials on the treatment of liver metastases (HEPAR 1) * and metastases from neuroendocrine tumors (HEPAR PLuS) ** were published in Lancet Oncology, a highly-regarded and impactful scientific journal.
The unique imaging properties of holmium-166 microspheres are well-fitted in the ongoing paradigm shift from a ‘one-size-fits-all’ approach to a more personalized approach in radioembolization. Both with conventional radioembolization and holmium-166 radioembolization, a direct relationship between tumor dose and treatment response was observed, as well as a relation between healthy liver uptake and liver effects. This shift emphasizes the need towards accurate pre-treatment planning, using all available options to ensure the tumor and healthy-liver dose are therapeutic and safe, before administration of the therapeutic microspheres themselves. Additionally, currently research is conducted at the Radboud academic hospital (Nijmegen, the Netherlands) on adaptation of the treatment dose during the radioembolization procedure itself, using real-time MR-guidance. This fits in a broader trend of personalized treatment and image-guided radiotherapy, with immediate tailoring and targeting of the radiation dose, aiming to improve both efficacy and safety.
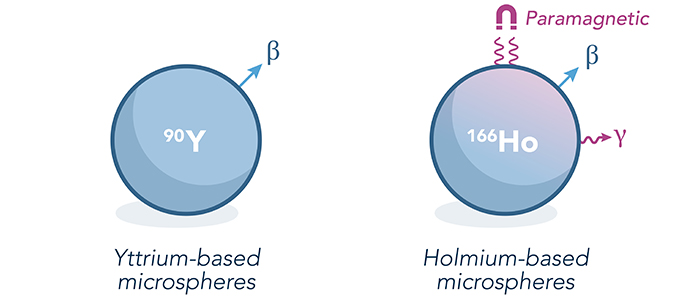
An illustration of the characteristics and the imaging properties of the two types of microspheres
The holmium platform is completed by a tailored software suite that supports physicians with pre-treatment planning and post-treatment evaluation of the holmium-166 radioembolization procedures. The treatment simulation product, in combination with the therapeutic microspheres and software package, can be seen as a holmium platform to support optimized, end-to-end radioembolization treatments for patients.
Holmium-166 radioembolization,
driving innovation in Interventional Oncology
Holmium-166 microspheres are currently manufactured and distributed by Quirem Medical, a medical devices company based in Deventer in the Netherlands. The company was founded in 2013 as a spin-off from the University Medical Center Utrecht with a mission to make holmium-166 microspheres available to hospitals around the world. In the summer of 2020, Quirem Medical became a wholly owned subsidiary of Terumo. It belongs to the Terumo Therapeutic Interventional Oncology (TIO) whose mission is to be the partner of choice to improve quality of life and survival of patients living with cancer by offering the best-in-class Interventional Oncology solutions.
The goal of the Terumo TIO business unit is to expand Terumo's interventional oncology portfolio with novel therapeutic solutions, and to provide a more comprehensive toolkit for treating cancer patients.
The Terumo TIO team is driving innovation in the interventional oncology field. Terumo TIO is working in close collaboration with numerous clinical partners globally to develop clinical evidence that supports a broader access to its solutions. It also actively develops more solutions for patients with cancers beyond the liver. Ultimately, Terumo TIO aims at developing truly personalized treatments to support patients with cancer.
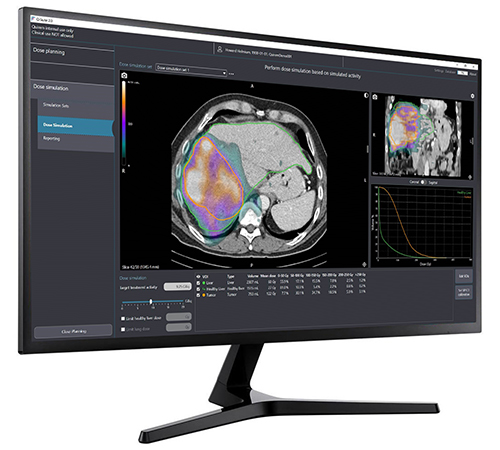
Imaging in treatment planning software
*
HEPAR 1: Smits ML, Nijsen JF, van den Bosch MA, Lam MG Lancet Oncol. 2012 Nov;13(11):e464
**
HEPAR PLuS: Braat AJ, Bruijnen RC, De Jong HW, Lam MG Lancet Oncol. 2020 Feb; 21(4);e561